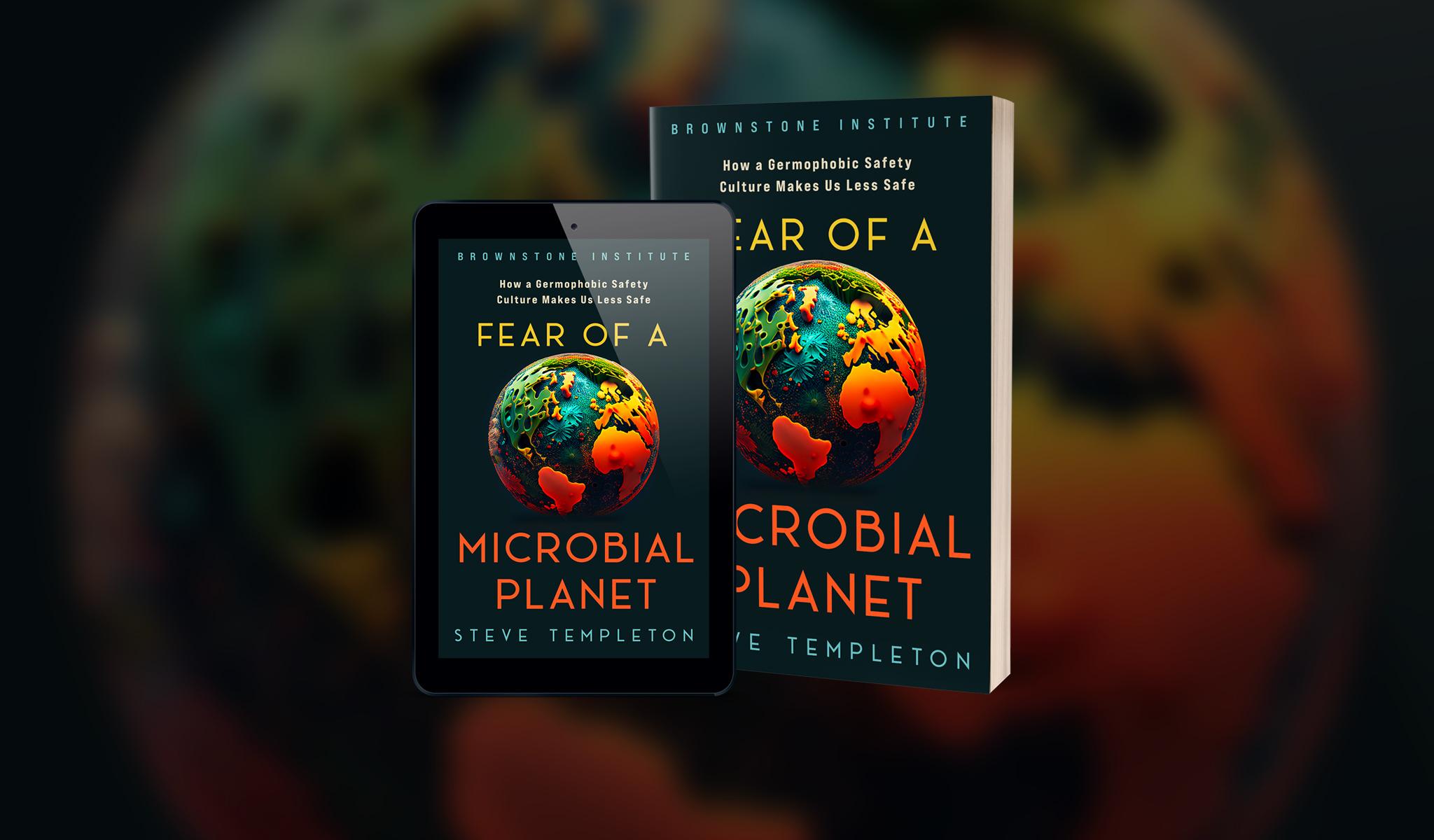
The following is an excerpt from Chapter 1 of Fear of a Microbial Planet: How a Germophobic Safety Culture Makes Us Less Safe.
When my sister goes into a hotel room for the first time, she takes a container of disinfecting wipes with her, and wipes down every surface that could have conceivably come into contact with a human being in the recent past. She doesn’t do anything else before this happens. No sitting down, no unpacking. Nothing.
“Why do you do that?” I asked her.
“You never know what or who’s been in there,” she answered.
That’s true of anywhere you go, I thought, but I didn’t press it further at that time. My sister is a germophobe, and I knew she wouldn’t be convinced by anything else her little brother might have to say, even if I was an infectious disease researcher. But maybe you will.
Germophobes Are Living in Denial
Germophobes (which could also be spelled germaphobes) live in denial because microbes are everywhere, and they cannot be avoided. There are an estimated 6×10^30 bacterial cells on Earth at any given time. By any standard, this is a huge amount of biomass, second only to plants, and exceeding that of all animals by more than 30-fold. Microbes make up to 90 percent of the ocean’s biomass, with 10^30 cells, equivalent to the weight of 240 billion African elephants. The very air you breathe contains a significant amount of organic particulate matter that includes over 1,800 species of bacteria and hundreds of species of fungi airborne in the form of spores and hyphal fragments. Some microbes can stay airborne for days to weeks, usually by hitching a ride on dust or soil particles. The sheer density in the air we breathe means we inhale thousands of microbial particles for every hour spent outdoors. Going inside isn’t much different, as indoor air is generally associated with the immediate outdoor environment, with differences due to ventilation and occupancy. It’s almost impossible to find any place, indoor or outdoor, that is completely sterile, although some places are dirtier than others.
If you are working in a musty, water-damaged basement without a protective respirator, stripping moldy drywall might expose you to hundreds of millions of aerosolized fungal spores very easily, irritating your throat, sinuses, and lungs. The leaves you raked in the fall, the ones you ignored for a while until they became a wet, brown mess until the weather finally became dry and warm, could have released a cloud of bacteria and fungi when you finally got around to raking or blowing them. And later, when you were relaxing in your hammock, you might have had a little cough. That was your lungs trying to get rid of all those microbes you stirred up and inhaled. But you probably got over it. Lungs are pretty good at clearing out most particles, even living ones.
Earlier, in the summer, when you went swimming in a lake, you were exposed to trillions of microbes the moment you hit the water. Bacteria and other single celled organisms had already bloomed in the warm, nutrient-rich water to astronomical levels for the summer season. Even if you thought you kept your mouth closed, you didn’t keep them completely out. No problem, you say, I’ll just swim in swimming pools, and avoid all of those germs. Yet swimming pools, despite containing antimicrobial levels of chlorine, may still contain fecal E. coli and Pseudomonas aeruginosa. Don’t even get me started on the kiddie pool. Did you think swim diapers stop much? Um, no. Poop, and the microbes that come with it, find a way.
All that bacteria in the lake and pool doesn’t just naturally live and multiply in the water. A significant amount originated in animals, including humans. We harbor trillions of bacteria on our skin, in our mouth, and in our guts. The pool doesn’t have microbes in it because the chemical treatments didn’t work, it has microbes in it because it has people in it. We are literally germ factories. It is all over us, inside us, and on everything we touch.
When I was in college, one local fraternity held a hot tub marathon fundraiser, where participants were sponsored to sit in hot tubs for as long as possible. Some did so for hours. In the next few days, many of them developed itchy, red, bumpy rashes with blisters surrounding hair follicles. Not surprisingly, all that time in the hot tubs turned them into large bacterial broth cultures, inoculated by fraternity guys and sorority girls in close proximity. The hot water, even chemically treated, couldn’t suppress the growth forever, and the bacteria, likely the skin-colonizing and rash-inducing Pseudomonas aeruginosa, grew exponentially. There wasn’t any sinister outside contamination. The source of all that Pseudomonas, without a doubt, was the people themselves.
Humans as Microbial Bioreactors
Our bodies are colonized by so many microbes that our cells (about 10 trillion total) are outnumbered by our microbial inhabitants by a factor of ten (about 100 trillion total). The microbiota of our bodies is incredibly diverse, with thousands of species of bacteria and fungi that collectively express 4.4 million genes, compared to our meager 21,000-gene genome. As science writer and ecologist Alanna Collen noted in her excellent primer to the human microbiota 10% Human, genetically we aren’t even 10 percent human, it’s actually more like 0.5 percent.
When and where do we get all of those microbes?
For anyone who’s witnessed a natural birth, it’s obvious the baby isn’t born in a completely clean environment. First of all, the mother’s vagina is loaded with bacteria, predominately of the genus Lactobacillus. You might recognize Lactobacillus from looking at the ingredient list of yogurt products, because it is often a major component. That’s why some crunchy midwifes tell pregnant women to rub yogurt on their vaginas if they think they might be getting a yeast infection. So, babies are exposed to yogurt bacteria? Nothing wrong with that! But that’s not all. Another common occurrence—women in labor might defecate. Because of intense lower abdominal and pelvic pressure, a woman in labor often starts to lose control and can sometimes push out everything. And as a result, the baby may be exposed to the mother’s fecal bacteria in addition to vaginal bacteria. If this exposure doesn’t happen at birth, it could also happen later in the hospital or household, as fecal bacteria are easily aerosolized/airborne and inhaled or swallowed. Either way, every health baby will ultimately be colonized by E. coli, Bacteroides, Clostridium, Staphylococcus, and Streptococcus species, just to name a few. If a mother is breastfeeding, the baby will also be exposed to additional Lactobacilli and Bifidobacteria.
Once a baby starts eating solid foods, her gut microbiota will adapt to the new sources of fiber, sugars, protein, and fats with increased diversity and a more “adult-like” microbiome. The adult microbiome is less dynamic as an infant in the first year of life, but adult microbiomes can still be disrupted by changes in diet, overall health, antibiotics exposure, or infection. I’ll get into more detail in Chapter 2 about how these changes can disrupt microbiomes and how they can be associated with modern health problems. But even with these disruptions, people are loaded with microbes, and are daily exposed to huge numbers of additional microbes at home, school, the office, or pretty much anywhere else on Earth.
Home is Where the Germs Are
When sequencing technology was also used to determine the microbial diversity in the air and dust of households and offices, the results were fascinating. Indoor microbes can be on surfaces or in the air as bioaerosols. Not surprisingly, the major source of indoor microbes and bioaerosols is the local outdoor environment. However, bioaerosols also come from animal and human occupants, due to breathing, shedding of skin cells, or using the toilet. Particles on surfaces can be resuspended in the air as bioaerosols by walking, vacuuming, cleaning, and even sleeping, since your bed is full of dead skin cells, fungi, and bacteria.
In any home or building with human occupants, species of human-colonizing bacteria are abundant. In fact, it is possible to predict whether a home is occupied by predominately males or females by their microbial profile, as higher percentages of males were associated with greater abundance of Corynebacterium, Dermabacter, and Roseburia species, whereas females were associated with increased Lactobacillus species. Whether a family had a cat or dog could also be determined by 16S rRNA sequencing. Dogs bring a higher diversity of bacteria, with 56 different types of bacteria compared to 24 from cats. Cats at least clean themselves, and spend much less time sniffing each other’s rear ends, so maybe that explains the difference.
What’s even more impressive is that, as the microbiota of more individuals were sequenced, it became obvious that each individual possesses a unique colony of microbes, as unique as a fingerprint. Although more or less stable through adulthood, these distinct microbiomes can be altered by factors such as diet, age, and hormones. Furthermore, genetically-related and cohabiting individuals tend to also have more similar microbial cohabitants. One study determined that when a family left a home, their microbes lingered for a few days, gradually decreasing to undetectable levels. This loss of microbial fingerprint could be used in the future by forensic scientists to help recreate a timeline of when a suspect vacated their home or hideout.
Unsurprisingly, the bathroom is the best place in a home or building to encounter microbes on surfaces or in the air. In a bathroom, something as simple as a toilet flush can generate bioaerosols containing billions of bacteria, some staying airborne for hours, long enough to travel to every nearby surface. Closing the lid can reduce the bacterial plume, but not as much as you might think. Even repeated flushing can’t completely eliminate the generation of fecal-bacteria-laden bioaerosols. As a result, when you walk into a restroom, you are going to inhale bacteria, and anything you touch will be covered with it. This does not bode well for your toothbrush. Yet somehow, you are still alive.
Aside from microbial exposures we get from our mothers and our immediate environment during and after birth, the most prominent sources of microbes that colonize our guts are determined by the food we eat. In newborn babies that are breastfed, breastmilk is both a source of bacteria and a food that those bacteria will love. Some bacteria in breastmilk may originate from the gut and are transported to the mammary glands by circulating immune cells, in addition to microbes that colonize the skin around the areola.
Also, when the baby drinks milk directly from the breast, some oral bacteria also join the milk-associated microbes on their journey to the gut. The types of bacteria transferred in this way are determined by the mother’s diet and the mode of feeding (e.g. either directly through the breast or indirectly through pumping). The infant microbiome changes when solid foods are introduced, until it begins to resemble a more-or-less stable adult microbiome around 2 ½ years of age. The results of numerous studies have shown that stages of early life are the most critical for the development of adult microbiomes.
Two Hours and Five Seconds to Gastrointestinal Doom
We all know people who are obsessed with the idea of keeping their food “clean.” Throwing any food away that sits out on a table longer than the time it takes to eat a meal or anything that falls on the floor have become pretty common first-world practices. There are few heuristics or shortcut rules that have become popular as a result, such as a the “two-hour rule” for leaving out food, and the “five-second rule” for eating food that has touched the floor. In my opinion the five-second rule is most advantageous for helping parents feel less guilty when their toddlers toss perfectly good food from their high chairs onto the floor. My toddler doesn’t give a rip about food hygiene, so why should I? Same goes for the two-hour rule—sometimes we get busy and forget the chili was on the cold stove all evening. Does that mean it’s still OK if we heat it up again? How did anyone survive before refrigeration?
If you are a food safety scientist or microbiologist, your job is to identify potential hazards in food storage and preparation that could lead to contamination and illness. This is mostly for industrial and commercial food production and preparation. It’s clear from anyone that inspects restaurants that they have a wide variety of procedures and some of them are better than others. Once a local inspector told me which restaurants she avoided (didn’t stop me, though, because I like one of the places too much). In her case, and in the case of food microbiologists, even the potential for a contamination is problematic. Of much less concern is relative risk, which is the likelihood that certain practices will lead to contamination and illness. Therefore, even the slightest risk might be considered a violation. To put it another way, even the slightest risk of inspectors looking like they aren’t doing their job could be a problem for them.
Over the years, this zero-risk thinking regarding food prep and storage has made it into the household. The two-hour rule is a good example. Most people wouldn’t even wait that long to throw food out. Yet, much of the worry over growth of pathogens in food left out for two hours is the result of some major assumptions. This includes assumptions that you start with a viable colony of one or more pathogenic microbes, that the food contains low amounts of salt and preservatives, a neutral pH, and that it is sitting at optimal temperatures above 80 degrees Fahrenheit (~27°C). The classic case of food poisoning used in microbiology classes is grandma making potato salad for the summer picnic, using her hands to mix it and thus inoculating it with skin-colonizing Staphylococcus aureus. Then it sits out on the picnic table all afternoon (way longer than two hours), and BAM, everybody gets food poisoning. That is certainly a good way to increase the chances of a family outbreak, but that’s the perfect storm, and a lot of things had to happen in that scenario in order to make everyone sick.
Cross-contamination can be a problem, especially if you are preparing something that will be eaten raw in the same place where you just cut up chicken. Even being clean with chicken has its limitations–the CDC cautions against washing it before you cook it, lest you create a bunch of bacteria-laden droplets around your sink. In reality, most food that is reasonably cooked is pretty safe, and four hours is a reasonable time to leave out most food at room temperature. As with everything, people are usually fine if they use common sense and clean up the messes they make in the kitchen.
Common sense also works for evaluating the five second-rule. The five second-rule states that if you pick up food before five seconds on the floor, it is OK to eat. Some studies and media reports have actually taken this seriously in order to point out that bacteria does in fact stick to your food no matter how long it is on the floor. But how useful is that? You will eat bacteria when your food touches anything that has come in contact with a non-sterile surface. More importantly, what are the chances the bacteria on that piece of food will be a pathogenic strain of bacteria or virus or deliver a sufficient dose to cause illness?
As I mentioned earlier, the microbes in an indoor environment more or less mimic those of the outdoor environment plus the microbiomes of its inhabitants, so chances are you are already swallowing or inhaling in much of that bacteria. Sure, if you use that piece of food dropped on the floor to prepare potato salad, and then leave it out in 100-degree heat all day, that might not be the best idea. Or, if you cut chicken the day before, and refused to clean up all of the juices that dropped on the floor, you might get a bigger dose of Campylobacter jejuni or Salmonella enteriditis than your body is going to be comfortable with. Otherwise, the chances you are going to die or even get sick from eating food that fell on the floor are pretty remote. Not zero, but closer to it than most people seem to think. Just don’t tell anyone I told you, and don’t let anyone see you do it.
The Theory of Bad Germs
The concept of a “healthy” microbiome has only been around for a few decades, but the concept of the “deadly germ that wants to kill us” has been around a lot longer. As a consequence of that historical imbalance, we still spend a lot of time on pathogenic microbes and less time on our how our normal microbial environment might keep out troublemaking bugs. As I have discussed, the technology that scientists use to study microbial ecology is fairly new. In contrast, the ability to isolate and culture a single disease-causing microorganism has been around for more than a century.
The concept of disease caused by microorganisms, known as germ theory, had to overcome several other competing theories. Some of the most popular were the miasma and filth theories. The miasma theory explained that diseases were caused by noxious gases in the atmosphere, released by rotting of organic matter. The very similar filth theory focused on contamination of water and air by human waste. Although these sound primitive by modern standards, they were championed by many mainstream scientists, even up until the 1930s. Even some terms we use today have origins in these theories, like malaria, which essentially means ‘bad air.’
It wasn’t until the late 19th century that Robert Koch presented his criteria, now known as Koch’s postulates, for demonstrating that a disease is caused by a specific, filterable microorganism. Like most scientific advances, Koch didn’t develop these ideas from scratch. Others were thinking along the same lines. But he succeeded where others failed with his clear explanation of how to reproduce his work and apply it to many different infectious diseases. Koch’s postulates state that you must be able to isolate an organism from an infected individual, grow it in culture, reintroduce it into a healthy animal, and reisolate and identify the microbe as identical to the originally isolated and suspected agent. He formed these postulates based on his work with anthrax, and further generated supporting data with tuberculosis and cholera.
Although the work done by Koch and others in isolating disease-causing bacteria set off an explosion in deadly germ-identification, other disease-causing agents like viruses remained hidden and unknown. They were too small to be visualized by light microscopes, and couldn’t be grown in culture without host cells to infect. One can imagine the scientists’ frustration when they observed diseases that were obviously infectious, but weren’t able to isolate the causative organism. A perfect example is the Spanish flu of 1918. Many researchers were eager to apply Koch’s postulates to discover the infectious agent from the lungs of flu patients. To complicate matters, flu patients with severe disease often develop pneumonia due to secondary bacterial infections. As a result, these organisms were initially believed to be causative organisms for influenza. More importantly, the same microbe couldn’t always be isolated from the lungs of flu patients. The result was a hot mess of conflicting evidence, and by the time a virus was identified as the causative agent of influenza, the pandemic was long over. I will get much more into influenza and other viruses in Chapter 3.
Once researchers understood the germ theory of disease, they could isolate many different disease-causing microorganisms and reintroduce them into experimental animals. But one thing that happened was that animals tended to be resistant to further challenges, due to an active immune response. By using experimental animals, the mechanisms of acquired immunity could be studied and applied to improve patient care through the development of antisera and vaccines that protect people from infection or reinfection. And that brings me to my favorite topic!
Immunology 101
I walked out of that my first undergraduate immunology class in 1994 sure that I was going to be an immunologist. That was over twenty-five years ago, and since that time I’ve introduced the immune system to many others as a teacher and mentor. The way I have often done it, using a classic example, goes something like this: the scenario starts when someone steps on a nail. My wife stepped on a protruding carpet nail in 2009 when we were staying at a less-than-perfect hotel while on a visit with her father in China. She was not happy about it because she was worried the nail might have introduced the bacterium Clostridium tetani into the soft tissue of her foot. If that happened, and the bacteria survived to multiply to sufficient levels, it would produce a nasty neuromuscular activity-enhancing toxin called tetanus toxin that would cause uncontrollable muscle contractions, most frequently presented as lockjaw.
Being an immunologist, I asked her something like, “But you’re vaccinated aren’t you? You were in the Peace Corps. They vaccinate you for everything.” She conceded that was true. “Then don’t worry about it. You’ll be fine,” I said confidently.
I could be confident because I understood the concept of immunological memory. The immune system is capable of activating cells that are specific to every conceivable pathogen, and once the infection has been cleared, some of those cells remain as memory cells, cells that are much more quickly and easily activated upon reinfection with the same or a similar bug. That’s the whole principle behind vaccination—we try to fool the immune system into thinking the body has been infected using parts of pathogens or a weakened pathogen to stimulate the same reaction and development of specific memory cells, without the risk of a serious primary infection.
If the early inflammatory response doesn’t prevent an infection, nearby tissue resident immune cells called macrophages will sense trouble. These cells hang out in our tissues waiting for a danger signal from an encounter with bacteria like C. tetani. Once activated, macrophages become very adept at phagocytosis (i.e. engulfing and degrading germs in intracellular bubbles called phagolysosomes), and are able to kill many invading microbes and remove host cells that die as a result of the infection.
In some cases, the early immune response won’t be enough to get rid of the small but significant amount of C. tetani or the toxin it makes after a person steps on a nail. That’s when the adaptive immune response kicks in. This starts about 4 days after the infection and peaks at about 10 days. The adaptive response starts when tissue-resident cells called dendritic cells (DCs) get activated with the same signals that activate other innate immune cells. Like macrophages, DCs phagocytose and break down pathogens into their component parts. However, once they become activated, they leave the infected tissue and migrate to a lymph node, where they directly interact with adaptive immune cells called T cells.
Since T cells are so diverse, only a few are activated during any given infection, and those activated cells frantically divide to produce millions of clones of themselves, dividing every 4-6 hours. They do this for several days in order to generate huge numbers of identical cells (that’s why an adaptive immune response takes time to get going). Many of the T cells that are activated in this way leave the lymph node and migrate to a site of infection, following chemical signals just like other immune cells.
At the same time, some T cells interact with other cells in the lymph node called B cells. B cells come from the bone marrow and can recognize parts of proteins outside the with receptors on their surface. B cells secrete a soluble form or their surface receptor that we call antibodies. Antibodies bind pathogens or proteins and promote their killing, uptake and degradation by macrophages. If a T cell recognizes the same part of pathogen, or “antigen,” then the T cell provides “help” to the B cell so that the B cell can make even stronger binding antibodies. Other T cells can kill infected cells, preventing the spread of an infection. Through these processes, the adaptive immune response generates a highly pathogen-specific response that is much more targeted, less damaging, and more regulated than the early innate inflammatory response.
Eventually, as the invading microbes and the toxins they produce are cleared by the adaptive immune response, the immune cells at the site of infection stop getting activation signals and start getting “cease and desist” signals. Most of those cells die and are picked up and degraded by macrophages who clean up the mess. Eventually, the tissue heals, dead skin and muscle cells are replaced, and things go back to normal.
But that’s not all that happens. In the lymph nodes and spleen, some of the activated T cells become memory cells. Memory cells can be activated and divide much more quickly if they ever see the same antigen again. In this way we have a memory of every infection we’ve had throughout our lives. Since vaccines mimic this response; we also have a memory of every vaccination we’ve ever had. Sometimes this memory wanes a bit, and we need to get another shot, or we would become susceptible to a mild(er) infection, but the help we get from memory cells during a reinfection or from a booster vaccination is better than starting from scratch. And that’s how the immune system keeps us alive in a world full of potentially deadly bacteria, fungi, and viruses.
If the immune system is so good at attacking bacteria, fungi, and viruses, why isn’t it always attacking the ridiculous numbers of microbes living around us, on us, and within us? Why doesn’t our immune system explode from all of the microbe-detection signals in our skin, lungs, mouth and gut?
It doesn’t do that because the immune system also has a property called immunologic tolerance, in which immune mechanisms are suppressed in order to avoid unnecessary collateral damage. Immune tolerance doesn’t just extend to our own self-proteins, it also extends to our non-threatening microbial environment. Tissues that have constant microbial exposure, like in our gut, are loaded with tolerance-inducing cells (called T regulatory cells) that help the immune system put a check on itself and prevent autoimmune disease.
But sometimes the immune system isn’t tolerant of what it should be, and people do get autoimmune diseases, or allergies, or have an inappropriate response to an infection. Interestingly, the incidence of these conditions is increasing everywhere in the developed world, because despite being surrounded by microbes, we are actually getting better at being “clean” than we realize.
Disclaimer
Some of the posts we share are controversial and we do not necessarily agree with them in the whole extend. Sometimes we agree with the content or part of it but we do not agree with the narration or language. Nevertheless we find them somehow interesting, valuable and/or informative or we share them, because we strongly believe in freedom of speech, free press and journalism. We strongly encourage you to have a critical approach to all the content, do your own research and analysis to build your own opinion.
We would be glad to have your feedback.
Source: Brownstone Institute Read the original article here: https://brownstone.org/